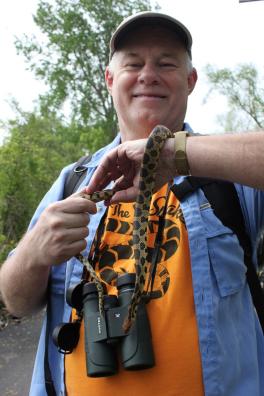
As a scientist, I found my passion studying snakes although I was trained to study birds — what happened? Two very different experiences were key in leading me to work with these extraordinary reptiles. First, as an undergraduate, I had a job checking nest boxes to collect data on baby birds. One day, to my immense surprise, I discovered a 3-foot-long black rat snake that had climbed inside the box and eaten the nestlings. I was furious about the fate of the poor birds and the loss, however a colleague I shared this with over lunch suggested that I should consider studying the predator and not just the prey. He was right. Second, one of the most iconic magazine covers I have ever seen was a close-up of the head of a highly venomous Malaysian pit viper on a cover of the journal Nature with the title “Natural selection bites.” That featured article described an amazing, yet infuriating, incomplete study which claimed to show how venom had evolved a molecular adaptation for feeding on different prey. As a graduate student, I had spent lots of time measuring the size of finch bills and relating this to the seeds they ate. The idea that you could study an adaptation at the molecular level was absolutely fascinating to me. As a scientist, I was hooked on the possibility of understanding how a complex adaptation could be “built” molecule by molecule, but at the time the methods for studying venom were very primitive and I tucked this idea away for future consideration.
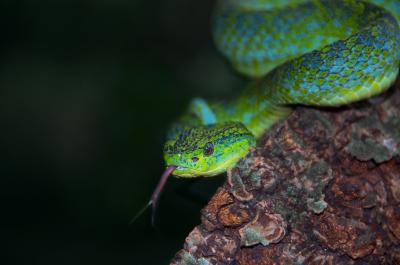
When I began working at Ohio State in 2001, I decided to follow up this inspiration by studying how venom evolved in a type of venomous snake called pit vipers — I call this line of work my research on the “Beak of the Snake.” Most recently, this work has involved a large collaborative research project between scientists in the U.S. at The Ohio State University, Florida State University and Clemson University in addition to Brazilian scientists at the Instituto Butantan in São Paulo, Brazil. Part of this work involved collecting data on the genetics and venom composition from hundreds of species of venomous snakes in North, Central and South America, building a phylogenetic tree showing the relationships among species, mapping information on venom composition onto the tree and comparing this variation to what the snakes eat. What we have found is that venom diversity is tightly correlated with prey diversity across many species of pit vipers, but that this relationship only holds for phylogenetic diversity in prey and not simply species diversity. We think the reason is that phylogenetic diversity of prey better accounts for the true diversity of venom-resistance molecules in prey than species diversity alone. In other words, in terms of how venom acts on prey, a mouse is a mouse — roughly the same type of venom molecules can act on closely related yet different species of rodents. However, for snakes that eat a more diverse diet — say mice and lizards — the targets for the venom are very different with each prey expressing distinct sets of resistance molecules. These require different types of venom molecules to subdue, making the whole venom more diverse. As a next step in this work, we are using an analysis of whole genomes from different species of pit vipers to understand how differences in venom molecules arise between species. Our results show that these differences are due to both changes in proteins produced by individual genes as well as variation in the abundance of these proteins due to changes in gene expression. Thus, venom as an adaptation is “made” through the evolution of venom protein sequences and variation in the amount that different sequences are expressed.
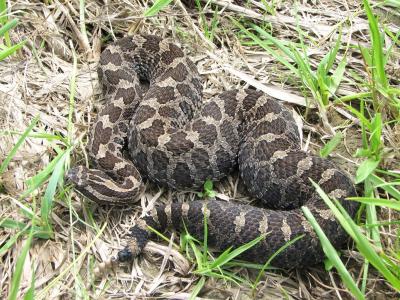
The other hat I wear is that of a conservation biologist. Specifically, I work on a second area of “snake”-based research has used diverse types of genetic information to gain knowledge useful for conserving rare species. Most of our work has focused on a small, timid, rare rattlesnake species named the Eastern Massasauga Rattlesnake, which is mostly found in small, isolated populations throughout the midwestern U.S. (including Ohio) and Ontario. Endangered species that live in small populations are at a higher genetic risk in two ways: (1) They can lose genetic variation that allows them to adapt to changes in the environment like those that result from climate change and (2) Genetic drift and inbreeding can allow bad mutations normally eliminated by natural selection to build up in populations. Understanding if either process occurs in a threatened species is essential for understanding if genetic issues are important to consider when developing management plans for species. To answer this question, we have been analyzing hundreds of whole genomes from individual Massasauga rattlesnakes from different populations across the U.S. and Canada. This work has been supported by the Ohio Division of Wildlife through the Ohio Biodiversity Conservation Partnership, which is a cooperative agreement between Ohio State and the Ohio Division of Wildlife. Surprisingly, we find that although Eastern Massasauga rattlesnakes show high levels of inbreeding, they have lower numbers of bad mutations present in their genomes compared to outbred rattlesnake species. We speculate that this is because these snakes may have always lived in small populations and as a result have been shedding bad mutations from their gene pool for a long time. This means that perhaps genetic issues may not be as important a consideration as we previously thought, and so the Ohio Division of Wildlife can focus their attention on ecological factors like habitat management. Future research in the next few years will tell us whether loss of “good” mutations is an issue for these snakes.